Understanding Plant Genotype: Role and Importance
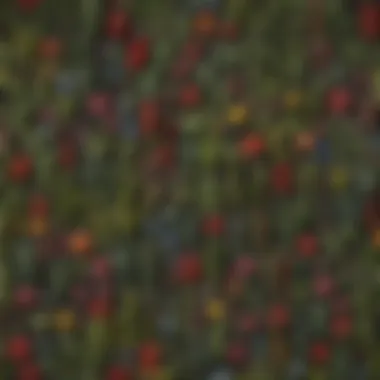
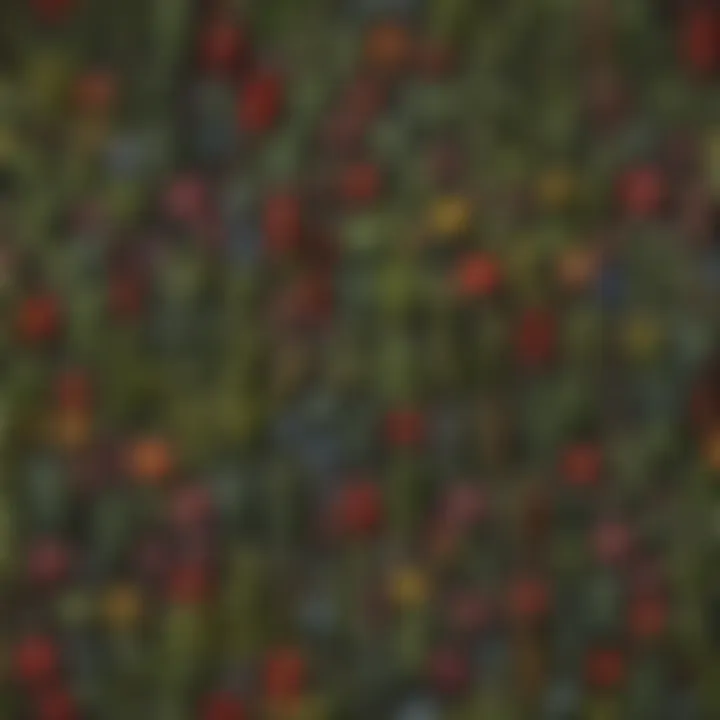
Intro
Plants form the backbone of our ecosystem and play a pivotal role in agriculture, horticulture, and agronomy. To enhance productivity and sustainability, understanding the concept of genotype is crucial. In simple terms, the genotype refers to the genetic constitution of a plant, impacting its potential traits and abilities. This section sets the stage for a deeper dig into how these genetic markers shape the life of a plant, its response to environmental conditions, and its functionality in agricultural practices.
Topic Overview
Definition of Key Terms
When we talk about genotype in plants, a few terms come into play, and understanding these can help simplify our conversation:
- Genotype: The genetic makeup of an organism, which includes the alleles inherited from its parents.
- Phenotype: The observable physical or biochemical characteristics of an organism resulting from the interaction of its genotype with the environment.
- Genetic Diversity: The range of genetic variation found within a population, essential for resilience against diseases, pests, and changing environments.
These terms are not merely academic jargon but relate directly to real-world applications in farming and plant development.
Relevance in Agriculture, Horticulture, and Agronomy
The genotype is a cornerstone in the field of agriculture. Farmers are increasingly embracing the significance of genetic diversity in their crops. This not only foster resilience in their yields but also aids in sustainable practices.
- With the rise in environmental challenges, such as climate change, understanding plant genotypes becomes central to crop improvement strategies.
- Moreover, horticulturists use knowledge of genotype to create hybrids that boast better flavors or shelf-life, which is crucial for marketability.
- Agronomists rely on genetic principles to develop crops that can thrive in specific geographical locations or soil types, tailoring their approaches to local conditions.
The interconnection between genotype and plant performance is clear; by harnessing genetic understanding, we can push towards a more sustainable agricultural future.
Current Trends and Innovations
Recent Advancements in the Field
A notable trend in plant genetics is the mapping of plant genomes. Advanced techniques enable researchers to identify specific genes linked to desirable traits — think drought resistance or increased yield.
- CRISPR Technologies: This gene-editing technique allows for precise modifications to a plant's genome, leading to improvements without introducing foreign DNA.
- Marker-Assisted Selection (MAS): This method aids breeders in selecting plants with preferred traits by using molecular markers, speeding up the breeding process.
Emerging Technologies and Practices
Innovations in biotechnology present fantastic avenues for enhancing plant resilience. Here are some noteworthy practices:
- Genomics: Scientists leverage sequencing technologies to gather comprehensive data on genetic variability among plant species.
- Participatory Breeding: Engaging local farmers in the breeding process ensures crops are tailored to their environmental needs, drawing from traditional knowledge.
Such strategies not only bolster crop production but also pave the way for eco-friendly and sustainable systems.
Practical Applications
Step-by-Step Guides or How-Tos
- Defining Goals: Establish what traits you want to enhance in your crops, taking into account local environmental factors.
- Choosing the Right Techniques: Select appropriate methods like CRISPR or MAS based on your goals and resources.
- Field Trials: Conduct trials to assess the effectiveness of the new traits in real-world conditions.
- Data Analysis: Collect and analyze data from these trials to make informed decisions moving forward.
Tips and Best Practices for Implementation
- Stay Informed: Keep abreast of the latest research and advancements in plant genomics.
- Collaborate: Foster relationships with scientists and agronomists to access genetic resources and expertise.
- Utilize Technology: Make use of agricultural tech, such as drone imaging and predictive analytics, to monitor plant health and genotype performance.
Finale
Delving into plant genotypes is not just an academic exercise. It is a vital asset in shaping the future of agriculture by aligning genetic science with sustainability efforts. Therefore, engaging with these concepts can empower farmers and agricultural professionals alike, driving forward efforts in crop improvement and resilience in an ever-changing world.
Prologue to Plant Genotype
The topic of plant genotype forms the backbone of modern agricultural understanding. In the context of improving crop yield and resilience, comprehending what genotype means is essential for farmers and agricultural enthusiasts alike. It is not merely about the visual attributes of a plant, but rather a deeper glimpse into its genetic blueprint, which governs every aspect of its existence from growth patterns to stress responses.
A plant's genotype comprises its specific hereditary information. This genetic data influences a diverse array of traits such as size, drought tolerance, and disease resistance. For farmers, having a firm grasp of genotype can lead to more informed decisions in crop management and breeding strategies, ultimately leading to enhanced productivity.
For instance, a farmer considering different seed varieties needs to understand that while two plants may look quite similar (in terms of phenotype), their underlying genotypes may dictate how they fare under various environmental conditions. As such, cultivating knowledge in this area enables targeted strategies that could yield significant benefits in both crop performance and sustainability.
Definition of Genotype
At its core, the term genotype refers to the distinct genetic constitution of an organism. In simpler terms, it’s like the plant's personal identity card, detailing the specific alleles it carries. These alleles are different forms of a gene, and they play a crucial role in determining how a plant will respond to environmental changes. For example, if a plant carries alleles for drought resistance, it stands a better chance of thriving during dry spells.
In a practical setting, think of genotype as the set of instructions that guides a plant’s development and adaptation. Advanced breeding practices, like hybridization or genetic modifications, are often focused on altering desirable traits by manipulating these genotypes. This understanding enables breeders to select for traits that meet the ongoing demands of climate change and food security challenges.
How Genotype Differs from Phenotype
While genotype encompasses the genetic makeup, phenotype refers to the observable characteristics that result from the interaction between genotype and environmental factors. For example, two plants with identical genotypes might exhibit different heights due to variations in soil nutrition or moisture availability. This distinction is pivotal for anyone involved in agriculture.
Here’s how they differ:
- Genotype: The inherited genetic information (alleles) within the plant.
- Phenotype: The physical expression of the genotype, influenced by environmental factors.
Understanding this difference allows farmers and researchers to better appreciate how much of a plant's traits are determined by genetics versus external conditions. When examining a crop's performance, getting to grips with both genotype and phenotype helps illustrate the full picture of how to optimize growth in varying situations.
"In agriculture, the line between genotype and phenotype can sometimes blur, but understanding their roles is key to unlocking the secrets of plant development and productivity."
By steering clear of misconceptions and recognizing the crucial roles these concepts play, one can more effectively navigate agricultural challenges, fostering greater success in crop production.
Historical Perspective on Plant Genetics
Understanding the historical context of plant genetics is akin to holding a map as you navigate through the intricate landscape of contemporary agricultural practices. This section aims to shed light on pivotal moments in the field of plant genetics, recognizing that the seeds of today's advancements were sown in the past. A grasp of earlier discoveries and theories allows farmers and enthusiasts to appreciate the evolutionary journey of plants and the scientific principles that guide modern agriculture.
Early Discoveries in Plant Genetics
The roots of plant genetics trace back to principles discovered by early botanists and scientists who laid the groundwork for our understanding of inheritance. Pioneers like Gregor Mendel are often the first to be mentioned in this context. In the mid-19th century, Mendel performed meticulous experiments with pea plants, observing how traits such as flower color and seed shape were passed down from one generation to the next. His work, while unrecognized during his time, eventually provided the foundation for what became known as Mendelian genetics.
Mendel's approach was systematic; he documented his findings in a manner that highlighted the predictability of inheritance. For instance, when he crossed purple and white flowered peas, he noted the appearance of the dominant purple in the first generation. The consistency of traits showed a pattern that others began to explore further. His observations laid the framework for understanding traits governed by discrete units – genes, as we call them now.
But beyond Mendel, earlier cultures had been practicing genetic selection long before science coined the term. Ancient farmers would have observed how crossbreeding different plant varieties often resulted in offspring with desirable traits. These ancient practices, while based on observation rather than genetics, show a fundamental understanding of selection that played a crucial role in agricultural development.
Mendelian Inheritance in Plants
Mendel’s laws of inheritance became critical frameworks that are still relevant today. The first law, the Law of Segregation, postulates that alleles separate during gamete formation, meaning that an organism carries two alleles for each trait, but passes only one to the offspring. The second law, the Law of Independent Assortment, states that genes for different traits are inherited independently of one another.
These principles had far-reaching implications for plant breeding practices. Breeders began to harness the power of Mendelian inheritance, particularly in developing strains that could better withstand diseases, pests, and varying environmental conditions. The concept of hybrids emerged, reshaping the agricultural landscape.
Moreover, Mendelian inheritance also encouraged the exploration of quantitative traits, like yield or height, which are significantly influenced by multiple genes. Such understanding led to advancements in selecting plants for desirable traits based on genetic predictions, propelling agricultural productivity.
In essence, Mendel’s insights were revolutionary. They steered the direction of plant genetics, giving birth to practices that farmers now utilize, often without realizing how deeply rooted they are in the historical discoveries of the past.
"Good farming practices are often rooted in the lessons of history. Those who ignore the past may very well sow the seeds of their own failure."
With this historical perspective, we begin to see the incredible evolution of plant genetics and its implications on modern agricultural practices.
The Relationship Between Genotype and Environment
The interplay between genotype and environment stands as a cornerstone in understanding how plants adapt and thrive in their surroundings. This relationship invites us to appreciate the nuances that make each plant unique, showcasing how genetic predispositions interact with the ever-changing climate and soil conditions. Such insights inform agricultural practices by explaining how certain traits can be enhanced or diminished based on environmental factors.
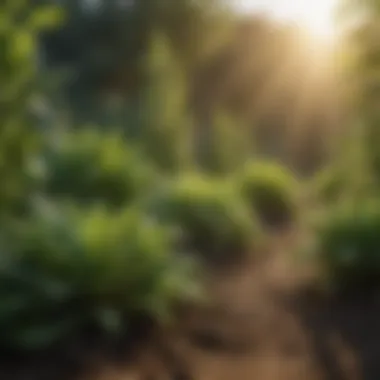
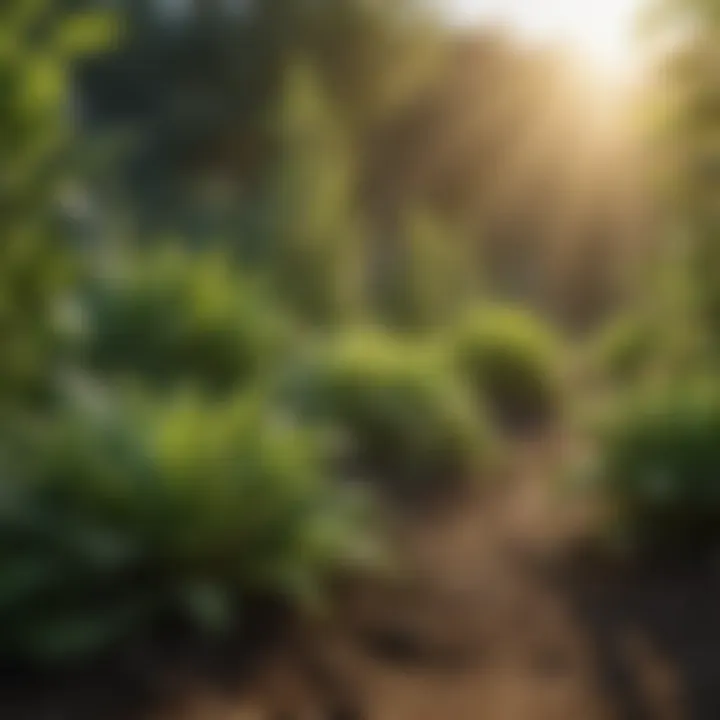
One critical aspect to consider is how genotype determines potential. Every plant carries a genetic blueprint that influences physical characteristics like size, drought tolerance, and disease resistance. However, these traits don’t exist in isolation. Instead, they emerge dynamically, influenced heavily by where and how a plant grows. The robust understanding of this dynamic helps farmers make better decisions regarding species selection and cultivation techniques, tailoring their approaches to capitalize on local conditions.
Genotypic Plasticity
Genotypic plasticity refers to a plant’s ability to alter its growth and development in response to environmental changes without genetic mutation. This is more than just survival; it’s adaptability in action. For example, a single genotype can produce plants with differing root depths based on soil moisture availability, effectively optimizing water usage.
- Key Benefits of Genotypic Plasticity:
- Enhanced Survival: Plants that can modify their traits are often more likely to endure extreme conditions, such as fluctuating temperatures or variable rainfall.
- Increased Crop Yields: By understanding which traits can be enhanced through plasticity, farmers can select varieties that produce better yields under local agricultural conditions.
By selecting genotypes with high plasticity, farmers can plan for better resilience against climate variability, ensuring sustainable production even in the face of future uncertainties.
Environmental Effects on Gene Expression
Environmental factors play a powerful role in gene expression, dictating which genes are turned on or off regarding specific traits. This phenomenon stresses the importance of not just understanding a plant’s genotype but also its ecological context. For instance, the presence of certain nutrients in the soil or varying temperatures can lead to different outcomes in the same plant species.
- Factors Influencing Gene Expression:
- Light Intensity: Plants exposed to higher light levels may express genes related to photosynthesis more robustly, thereby improving energy capture.
- Soil Properties: The nutrient composition of soil can lead to significant differences in growth rates and stress tolerance.
"Understanding how the environment affects gene expression allows agronomists to tailor breeding programs to enhance traits that lead to more robust plants in specific locales."
Through the lens of gene expression, it becomes increasingly clear that to optimize growth and yield, the cultivation strategies must consider environmental interactions. This approach merges the idea of genotype with practical applications, helping farmers and botanists alike harness the full potential of plant genetics in sustainable farming practices.
Methods of Genotyping Plants
Genotyping plants plays a pivotal role in understanding genetic makeup and variability, which is fundamental for successful breeding programs, conservation efforts, and agricultural advancements. Through genotyping, it's possible to uncover genetic traits that influence a plant's ability to adapt to its environment or resist diseases. The choice of method can greatly impact the efficiency and effectiveness of the research or agricultural initiative.
Traditional Techniques
In the realm of plant genotyping, traditional techniques lay the groundwork. These methods, while often more labor-intensive and subject to limitations, offer valuable insights into the genetic framework of plants. Common traditional methods include:
- Morphological Assessments: Observations relating to the visible traits of plants.
- Isoenzyme Analysis: This technique examines the different forms of enzymes, which can help identify genetic variations.
- Chromosomal Mapping: By studying the arrangement of chromosomes in cells, researchers can pinpoint genetic markers associated with specific traits.
Although these methods might lack the precision of modern techniques, they can still provide a foundational understanding of genetic diversity. They are often more accessible for smaller-scale studies where high-tech equipment isn’t readily available.
Modern Genotyping Techniques
Moving forward into today's age, modern genotyping techniques utilize advanced technology to provide more accurate, rapid, and comprehensive data. Here are some significant modern methods:
PCR (Polymerase Chain Reaction)
PCR is a widely-used technique in molecular biology for amplifying small segments of DNA. This aspect of PCR is vital for studying specific genes or mutations within the genetic material of plants.
One key characteristic of PCR is its ability to produce millions of copies of a particular DNA sequence from a small initial sample. This feature makes it a powerful choice in plant genomics. The advantage of PCR lies in its precision and the speed at which it can generate results, allowing researchers to identify genetic variations promptly. However, it requires a certain level of technical expertise and can be sensitive to contamination, which is a downside.
SNP Analysis
SNP analysis focuses on single nucleotide polymorphisms, which are variations at single positions in DNA sequences among individuals. This aspect of SNP analysis is essential for understanding genetic variations that could influence important traits in plants.
The standout feature of SNP analysis is its high-resolution genotyping capabilities. It's widely appreciated in modern research because it allows for a large-scale analysis of genetic diversity across populations. The upside is the richness of data that can be derived from SNPs, but it might also necessitate robust data management systems due to the volume of information generated.
Whole Genome Sequencing
Whole genome sequencing allows the complete sequencing of an organism's DNA, granting an in-depth look at its genetic blueprint. This aspect aids in comprehensive studies of plant genetics and evolution.
What makes whole genome sequencing remarkable is its ability to identify all genetic variations simultaneously, providing an exhaustive overview of genetic resources. This technique is beneficial for creating detailed genetic maps and understanding complex traits influenced by multiple genes. However, it can be costly and requires substantial computational resources for data analysis, which can be prohibitive for some researchers.
"The methods we use to understand plant genotypes not only enhance crop improvement but also contribute to biodiversity and ecosystem health."
In summary, the exploration of traditional and modern methods for genotyping plants reveals a spectrum of tools available to researchers and agricultural professionals. Choosing the appropriate method influences research outcomes, breeding programs, and ultimately, the future of sustainable agriculture.
Genetic Diversity in Plant Populations
Genetic diversity refers to the total number of genetic characteristics in the genetic makeup of a species. In the realm of plants, genetic diversity is paramount for numerous reasons, especially as agriculture faces ever-changing challenges such as climate change, diseases, and pests. The concept encompasses not only the sheer variety of alleles within a single population but extends to the differences between various populations of the same species. This diversity serves as the backbone of resilience in plant populations, influencing everything from adaptability to productivity.
Genetic diversity within plant populations can lead to several benefits which, in turn, have far-reaching implications for agriculture:
- Adaptability: Diverse genetic traits allow plant populations to withstand adverse environmental conditions. For instance, if a particular strain of a crop is susceptible to drought, a genetically diverse population may contain varieties that thrive in such conditions, thus ensuring sufficient yield even under stress.
- Pest and Disease Resistance: When genetic diversity is robust, the likelihood of entire populations succumbing to a single pest or disease diminishes. This concept is often depicted using the adage, "Do not put all your eggs in one basket." A variety of genetic traits among plant populations can lead to mixed resistances, helping ensure longevity and sustainability in crop production.
- Ecosystem Stability: Thriving ecosystems depend on genetic diversity to maintain balance. Pollinators, soil microbes, and a host of other organisms rely on various plants for their survival. A lack of genetic diversity can disrupt these relationships, leading to a cascade of ecological consequences.
Moreover, preserving genetic diversity plays a crucial role in local agriculture and food security. As mentioned earlier, diverse genetic makeup enhances resilience, making populations less vulnerable to sudden changes in climate or pest outbreaks. For farmers and agricultural stakeholders, this presents an opportunity to secure their livelihoods against future uncertainties.
Importance of Genetic Diversity
The significance of genetic diversity transcends mere survival; it's a cornerstone for sustainable agricultural practices. When contemplating the importance of genetic diversity, one must consider various facets, including:
- Enhanced Crop Yield: Diverse genotypes can lead to improved traits such as growth rates, yield stability, and nutritional content. Essentially, this results in better food security for growing populations.
- Resource Optimization: Cultivating a variety of crops with different genetic backgrounds allows farmers to make full use of available resources, such as soil and water. This aligns with sustainable practices, as it mitigates over-reliance on a single crop type.
- Cultural Significance: Genetic diversity helps preserve indigenous varieties that might have personal or cultural importance. These crops often come with unique flavors or characteristics that are integral to local cuisines.
An example that illustrates both the importance and the benefits of genetic diversity is the case of the potato. During the Irish Potato Famine in the 19th century, the reliance on a few genetically similar potato varieties led to widespread crop failure due to blight. Had there been greater genetic diversity in potato crops during that time, the impact may have been far less severe.
Conservation of Genetic Resources
Conserving genetic resources is increasingly viewed as a critical venture, especially with the rising pressure of industrial agriculture and monoculture practices. Here are several essential aspects of conservation efforts:
- Seed Banks: Organizations such as the Svalbard Global Seed Vault play a vital role in preserving genetic material. They house a wide variety of seeds, ensuring that genetic diversity is safeguarded against catastrophic events that could endanger plant species.
- In Situ Conservation: This approach involves preserving species in their natural habitats. By maintaining ecosystems where diverse plant species can flourish naturally, it's possible to sustain both genetic and ecological diversity.
- Restoration Efforts: Initiatives focused on restoring degraded land can also support the reestablishment of genetically diverse plant populations, protecting them from extinction and enabling them to thrive in their habitats again.
Protecting genetic diversity is not merely an ethical responsibility but essential for future food security. With climate stresses looming, evaluating strategies that prioritize diversity over uniformity stands to benefit not just agriculture but the environment as a whole. By fostering a more extensive genetic pool, we enhance the potential for adaptability, resilience, and sustainability well into the future.
Breeding Strategies Based on Genotype
Breeding strategies rooted in genotype are fundamental to enhancing plant qualities and ensuring agricultural sustainability. By understanding a plant’s genetic makeup, farmers and researchers are better equipped to develop varieties that are not only high-yielding but also resilient to pests, diseases, and varying environmental conditions. The role of genotype in breeding is akin to having a treasure map; it helps pinpoint the traits that are crucial for improving crops.
When we dive into these breeding strategies, it's important to focus on a few specific elements. Firstly, it emphasizes the goal of achieving precise selections in breeding programs, with clear benefits such as increased crop productivity and disease resistance. Furthermore, it invites innovative considerations, whereby integration of genetic information into breeding practices can accelerate development, turning the tide in agriculture toward more sustainable practices.
Conventional Breeding Techniques
Conventional breeding techniques have long served as the cornerstone of agricultural improvement. This approach leans heavily on the selection of parent plants with desirable traits for cross-pollination. Over generations, it leads to offspring that inherit these traits. This method, known as selective breeding, is akin to sculpting a block of marble. With each generation, careful chiseling reveals a statue defined by the best features.
For instance, if farmers desire a variety of corn that yields more and is resistant to certain diseases, they will select plants that showcase these features for breeding. It can often involve:
- Phenotypic Selection: Choosing based on observable traits, such as height or color, which provides immediate feedback on plant performance.
- Hybridization: Crossing different plant varieties to produce hybrids, often resulting in enhanced vigor and yield.
This age-old technique is reliable but can be slow due to the time taken for generations to pass. Therefore, while it has proven effective, it also presents limitations, particularly in the context of rapid change and the need for flexibility in crop adaptation.
Molecular Breeding Approaches
In contrast to conventional methods, molecular breeding approaches have emerged as a frontier in agricultural science, leveraging the power of genetic information. These techniques utilize molecular markers associated with specific traits, enabling breeders to select plants based on their genotype rather than just phenotypic characteristics. It’s like trading a compass for GPS—much more precise and faster.
Molecular breeding includes a variety of sophisticated practices, which often include:
- Marker-Assisted Selection (MAS): This technique allows breeders to identify plants carrying desired alleles without waiting for the plants to mature. It simplifies the selection process significantly by focusing on the genetic markers rather than waiting for the end result to show in physical traits.
- Genomic Selection: Here, a large number of molecular markers are used to predict the performance of untested lines based on their genetic profiles. This method is faster and requires fewer resources compared to traditional breeding.
- Intracellular Genetic Engineering: Here, traits can be introduced directly at the molecular level, with approaches such as CRISPR-Cas9 being a part of this modern breeding toolkit.
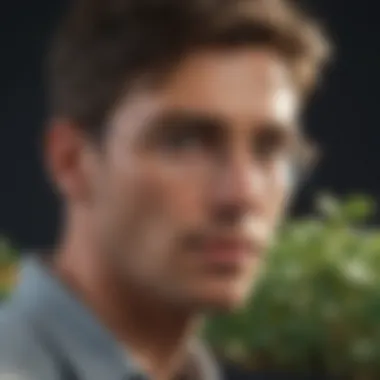
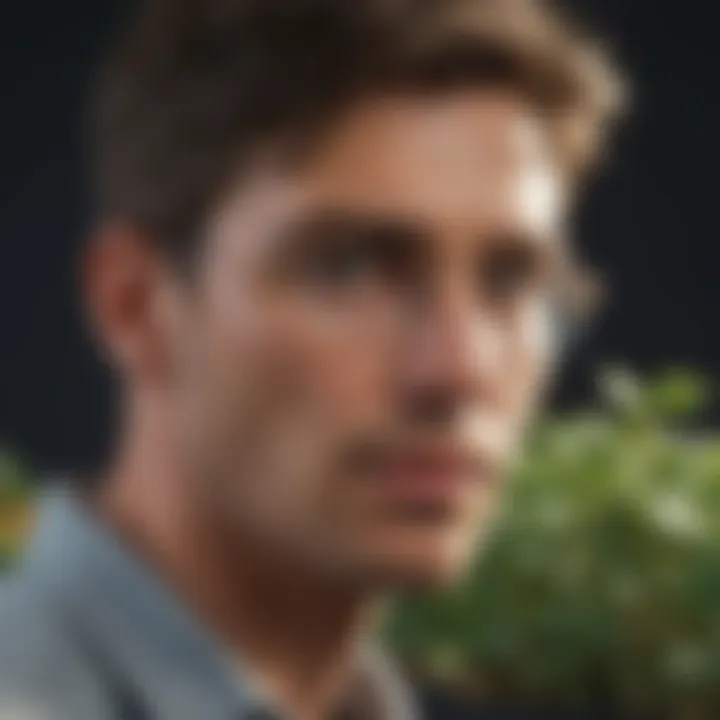
Overall, molecular breeding significantly speeds up the process of developing new plant varieties, which ultimately supports the need for more resilient crops to meet the challenges posed by changing climates and growing populations.
"The future of agriculture is not just in the fields but within the genetic codes we unlock."
Applications of Genotyping in Agriculture
The integration of genotyping into agricultural practices has become a linchpin in improving crop productivity and resilience. By delving deep into the genetic make-up of plants, farmers and researchers can make informed decisions that are grounded in science rather than guesswork. The applications of genotyping touch upon various facets of agriculture, such as crop improvement, stress resistance, and overall sustainability.
One significant aspect is that genotyping allows for the identification of desirable traits in plants. This ability is vital when developing new cultivars, as it ensures that specific phenotypic characteristics, like yield, disease resistance, or drought tolerance, can be enhanced or introduced.
"A targeted approach in crop improvement is not just a luxury; it’s a necessity in modern agriculture."
Crop Improvement Programs
Crop improvement programs increasingly rely on genotyping as a core component of their strategies. Traditional breeding often takes years, if not decades, to yield desirable results. But with genotyping, the process can be accelerated significantly. By identifying alleles associated with advantageous traits, breeding programs can select parent plants that carry these alleles, making it easier to produce offspring that meet farmers’ needs.
These programs often utilize molecular markers derived from genotyping, which serve as indicators for specific traits. For instance, breeders can use single nucleotide polymorphism (SNP) markers to track resistance to diseases like powdery mildew or fungal infections. This information is invaluable, as early generations of plants can be screened for these traits, vastly reducing the time and resources spent on less promising individuals.
Enhancing Stress Resistance
In today's world, where climate change is wreaking havoc on agricultural systems, enhancing stress resistance through genotyping presents a viable pathway to safeguard crops. Stressors like drought, salinity, and extreme temperatures can devastate yields. Through genomic analysis, scientists can identify stress resilience genes in various plant species. Once identified, these genes can be introgressed into commercially valuable cultivars using advanced breeding techniques.
Understanding the genetic basis of stress resistance provides multiple advantages:
- Localized Adaptation: Plants can be tailored for specific environmental conditions in different regions.
- Increased Yield Stability: Breeding stress-resistant varieties helps ensure higher productivity, even under adverse conditions.
- Sustainable Practices: By reducing the need for chemical inputs like fertilizers or pesticides, sustainable agriculture practices can flourish.
Overall, the applications of genotyping in agriculture are profound and multifaceted. They provide a roadmap for developing crops that not only meet global food demands but also remain resilient in the face of environmental challenges.
Genotype-Environment Interactions
Understanding the interactions between genotype and environmental factors is cructial for the development of crops that not only yield well but also adapt efficiently to varying conditions. Genotype—representing the genetic makeup of a plant—plays a significant role in how a plant responds to its environment. It’s not just about what a seed has in its DNA; it’s about how that DNA interacts with environmental pressures, which can dictate everything from growth rates to disease resistance. Therefore, exploring these interactions leads to opportunities for optimizing crop production and ensuring resilience against climate variability.
Impact on Crop Yields
The genotype of a plant significantly influences its potential crop yield, largely by determining how effectively it can exploit the conditions provided by its environment. There are several layers to consider here:
- Nutrient Uptake and Utilization: Certain genotypes are engineered or selected for superior nutrient uptake systems. For instance, some varieties of rice can absorb nitrogen more efficiently, leading to higher yields when grown in nutrient-poor soils.
- Water Efficiency: Drought-resistant genotypes can maintain yield levels during periods of low rainfall, signifying that understanding which traits confer this advantage can be paramount for farmers in arid regions.
- Response to Pests and Diseases: The capability of a plant to withstand pest attacks is also largely genotype-dependent. Specific traits may favor the development of natural resistance, making them essential for sustainable agriculture.
This complex relationship between genotype and yield can be seen in several studies demonstrating that improvement in crop varieties has been closely tied to advances in understanding these interactions.
"The complexity of genotype-environment interactions is akin to a dance—each step influences the next, often determining the success or failure of the entire performance."
Adaptation and Resilience
As climate change presents new challenges, crop adaptation becomes vital. Plants must not only survive but thrive in changing conditions, making resilience a key focus in breeding programs:
- Phenological Adaptation: Certain genotypes adjust their growth stages based on environmental signals. For instance, some legumes can alter flowering times to match rainfall patterns, ensuring that they reproduce effectively.
- Stress Response Mechanisms: Genotypes with enhanced capabilities to cope with stresses—like heat tolerance or salt resistance—can sustain productivity under adverse conditions. The development of such varieties is critical as environmental stresses become more common.
- Biodiversity in Genotype Pools: Maintaining a diverse genotypic pool within a species can be beneficial. It allows crops to adapt through natural selection over time, promoting resilience against unforeseen climatic changes or pest outbreaks.
The significance of genotype-environment interactions goes beyond immediate agricultural yields; it lays the groundwork for future advancements in crop science. Breeding programs that focus on understanding these intricacies will pave the way toward more sustainable practices in agriculture.
Insights from Genomic Studies
Genomic studies have become a cornerstone in understanding genotype and its implications for plant biology today. The insights garnered from these studies not only improve our grasp of the genetic underpinnings of plants but also pave the road for innovative agricultural practices. Using genomic data, researchers can pinpoint key traits that enhance resistance to diseases, adapt to shifting climates, and optimize yield potential. This is of utmost importance in an era where food production needs to keep pace with a growing global population while also considering sustainability.
Genomic Selection in Plants
Genomic selection represents a game-changing approach in plant breeding and genetics. The focus here is on harnessing genomic information to predict an individual's breeding value based on the genotype. By using genomic data, plant breeders can make informed decisions, significantly shortening the breeding cycle compared to traditional methods. This precision allows for more rapid advancements in creating new cultivars.
One of the benefits of genomic selection is its ability to facilitate the identification of favorable genetic markers linked to critical agronomic traits. For instance, traits like drought resistance or nutrient efficiency can be selectively enhanced. This means that, rather than waiting years to see if a specific crossbreed yields the desired result, the outcomes can be anticipated faster, reducing the guesswork and improving success rates.
Additionally, genomic selection encourages a more personalized approach to farming. Farmers can use specific genomic insights to choose crop varieties that best suit their local environmental conditions, potentially leading to better yields with lower resource inputs. With this technology, it becomes increasingly feasible to develop crops that align with both profitability and environmental stewardship.
"Genomic selection is not just a tool; it’s a paradigm shift that redefines what is possible in agricultural innovation."
Functional Genomics Applications
Functional genomics, another vital aspect stemming from genomic studies, aims to understand gene functions and interactions at a holistic level. This is particularly important in agriculture, as plants interact with a variety of environmental factors. Functional genomics can shed light on how plants respond to stressors, including abiotic stress like salinity, drought, and extreme temperatures.
Through techniques like gene editing and transcriptomics, researchers can explore the roles of specific genes in particular traits, making it easier to manipulate them for desired outcomes. For instance, researchers might identify a gene that increases root depth, allowing a plant to access water from deeper soil layers during drought conditions. By tapping into this genetic potential, we can engineer more resilient crops, which is critical in the face of climate variability.
Moreover, functional genomics extends into fields such as phytochemistry, where researchers can unlock the biochemical pathways that contribute to valuable traits like pest resistance or enhanced nutritional profile. This opens doors for both increasing food quality and reducing dependency on chemical inputs, a benefit not just for farmers, but also for consumers and the environment alike.
Sustainability and Genotype Innovations
In the face of climate change and ever-increasing agricultural demands, sustainability in agriculture has become more than just a buzzword; it is a necessary approach for ensuring the survival of ecosystems and food security. The interplay between genotype and sustainable agricultural practices results in a more resilient farming system. By understanding and applying genotype innovations, farmers can enhance productivity while protecting the environment.
Integrating Genotype into Sustainable Practices
Integrating genotype into sustainable practices involves recognizing how genetic traits can be optimized to adapt crops to specific environmental conditions. This is where crop breeding and biotechnology come into play. Here are some key elements that embody this integration:
- Selection for Resilience: By focusing on genotypes that exhibit drought resistance or pest tolerance, farmers can plant crops better suited to their local environmental challenges. This not only boosts yield but reduces the need for pesticides and excessive irrigation.
- Conservation of Resources: Employing genotyping methods enables the identification of plant varieties that efficiently utilize available nutrients and water. This leads to sustainable land use and helps preserve soil quality over the long term.
- Community Engagement: Involving local farmers in genotype selection fosters collaboration and enhances the adoption of sustainable practices. The knowledge of community-based breeding can lead to more tailored solutions that fit specific regional challenges.
"Sustainable agriculture is not only about the crops we grow; it's about the communities we build around those crops."
Moreover, advances in modern genotyping techniques, like genome sequencing and SNP analysis, allow for a more precise approach to identifying desirable genetic traits. This precision enables farmers to make informed choices about which plants to breed, making agricultural practices more sustainable.
Future Trends in Plant Genotyping
The future of plant genotyping looks promising as innovations continue to emerge. Here’s what we can expect:
- CRISPR Technology: This cutting-edge gene-editing tool isn't just a laboratory gimmick; it's already being integrated into practical farming applications. With CRISPR, it's possible to create crop varieties that can withstand climate extremes or have improved nutritional profiles without altering the genetic foundations of the plants.
- Plant Phenotyping Advances: Utilizing advanced imaging and AI technology, phenotyping is becoming more efficient. This allows for better understanding how genotypes express themselves under various environmental conditions, leading to more informed selections in breeding programs.
- Digital Agriculture: The rise of data analytics in agriculture means genotypes can be continuously monitored and evaluated based on field performance. This real-time data will enable farmers to be more adaptive and responsive to market and environmental changes.
In summary, the integration of genotype innovations into sustainable practices is integral for future-proofing agriculture. Advances in genotyping will lead to crops that are not only resilient but also more aligned with our environmental and societal goals.
Ethical Considerations in Genotyping
Genotyping in plants is not merely a scientific endeavor; it intersects significantly with ethical principles that warrant thorough examination. This section addresses the ethical considerations in genotyping, emphasizing the impacts of genetically modified organisms (GMOs) and the relevant regulatory frameworks. Engaging with these ethical aspects is crucial for agronomists, researchers, and policy-makers alike, as it informs decisions that affect biodiversity, food security, and public health.
Impacts of Genetically Modified Organisms (GMOs)
The advent of GMOs marks a significant milestone in agricultural practices. On one hand, they present unprecedented possibilities in crop improvement. For instance, crops like Bt cotton, engineered to express a toxin that is harmful to specific pests, have led to substantial increases in yield and reductions in pesticide usage. On the flip side, the use of GMOs raises critical ethical concerns such as:
- Biodiversity loss: The reliance on a narrow range of genetically modified crops can lead to reduced genetic diversity, which is essential for ecosystem resilience.
- Health implications: The long-term health effects of consuming GMOs are still under investigation. There are concerns that potential allergens or harmful substances might emerge.
- Economic equity: Smaller farmers might find themselves at a disadvantage if they cannot afford GM seeds, leading to corporate monopolies in the agricultural sector.
The potential for GMOs also invites a more philosophical debate about what is considered "natural" and who decides what modifications are acceptable. While biotechnology holds great promise, the balance between innovation and ethics is delicate and requires ongoing dialogue among stakeholders.
Regulatory Frameworks and Policies
As the landscape of plant genotyping continues to evolve, so too must the regulatory frameworks that govern it. These regulations are designed to ensure that the development and use of genetically modified plants align with ethical standards and public safety. Key components of these frameworks include:
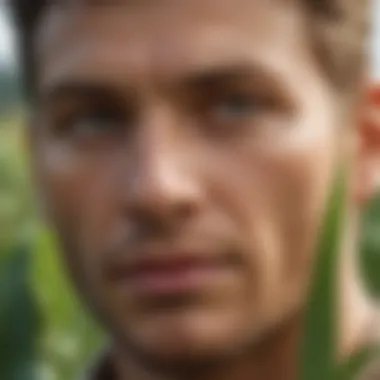
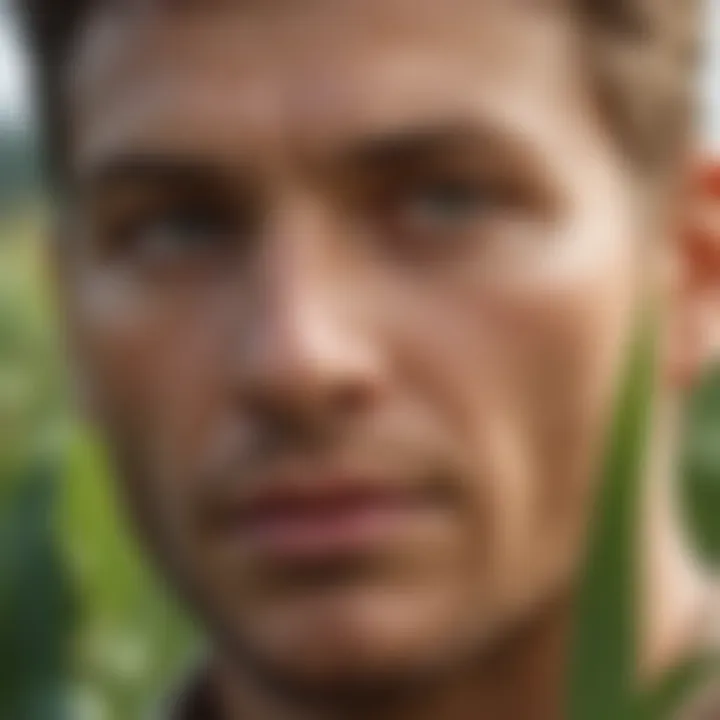
- Safety assessments: Before GMOs can reach the market, they undergo rigorous evaluations to ascertain their safety for human consumption and environmental impact. This often involves long testing periods where potential risks are identified and mitigated.
- Transparency and labeling: Many countries require that GMOs be labeled, giving consumers the right to make informed choices about the foods they consume. This is a crucial aspect of ethical responsibility, fostering trust between consumers and producers.
- International guidelines: Organizations such as the Codex Alimentarius advocate for international food safety standards, helping to harmonize regulations among different countries. This is especially important in a globalized food market.
As the precedents set today will affect generations to come, it is imperative that we approach the ethical considerations of plant genotyping with a sense of responsibility and foresight.
In light of these ethical considerations, ongoing stakeholder engagement, scientific research, and transparent policy-making will play a critical role in shaping the future of plant genotype research. Only by fostering a comprehensive understanding of these issues can we navigate the complex terrain of agricultural innovation responsibly.
Challenges in Plant Genotyping
Genotyping plants offers a wealth of benefits,from improving crop yields to enhancing resilience against environmental stresses. Yet, it doesn’t come without a fair share of hurdles. Understanding these challenges is crucial, as they play a significant role in the success and implementation of genotypic studies in agriculture.
Technical Limitations
When one thinks of technical limitations in plant genotyping, several elements might spring to mind. First and foremost, the accuracy of the methods used. Traditional techniques, while foundational, often fall short when it comes to high-throughput or complex genome analysis. For instance, when analyzing a plant species with a massive genome, such as wheat, the whole process may become cumbersome and time-consuming. The ability to handle complex genetic structures poses another layer of difficulty.
Furthermore, not all genotyping techniques are universally applicable; some may work wonders for one crop but turn into a headache for another. This middle ground can lead farmers and researchers down blind alleys.
Data Management and Analysis Issues
Once the technical pieces are in place, the challenges shift towards data management and analysis. Plant genotyping produces copious amounts of data, which can be quite overwhelming. Imagine trying to cook a feast for a family reunion with a recipe that has a dozen complicated steps; that’s how overwhelming it can be to manage raw genetic data.
Moreover, ensuring that this data is not only stored securely, but also analyzed effectively, is a different ball game altogether. Limited software tools or inadequate training on existing ones can stymie progress and lead to misinterpretations. For example, a farmer may end up cutting out potential high-yielding plants from the breeding pool because of erroneous data analysis.
In summary, tackling challenges in plant genotyping is akin to navigating a minefield. It requires a careful approach and a sound understanding of both the technical and analytical aspects to ensure that the benefits far outweigh the roadblocks.
"Understanding the obstacles is the first step in overcoming them."
By addressing these challenges head-on, the agricultural community has the potential to reap substantial rewards, paving the way for innovations that can enhance food security and sustainability.
The Future of Genotype Research
The trajectory of genotype research is crucial for shaping the future of agriculture. With evolving challenges such as climate change, declining biodiversity, and food insecurity, understanding genotype paves the way for innovative solutions in agricultural practices. When we comprehend the genetic basis of plants, we unlock doors to breeding more resilient crops. Impressive advancements in technologies promise to refine our capabilities in identifying and manipulating these genetic traits.
Emerging Technologies
In the realm of plant genotype research, several emerging technologies are making significant headway.
- CRISPR-Cas9 Technology: This revolutionary gene-editing tool allows for precise alterations in the plant genome. Researchers can enhance traits such as drought resistance or nutritional content.
- Next-Generation Sequencing (NGS): NGS has transformed our approach to genotyping by providing comprehensive insights into genetic structures. With faster results and lower costs, it allows for large-scale genotyping and analysis.
- Phenotyping Platforms: Advanced imaging technologies and sensor-based systems track plant growth and responses to various stimuli. Such data can correlate genetic information with real-world plant behavior, bridging genotype and phenotype effectively.
- Bioinformatics: The use of sophisticated software tools to analyze vast amounts of genetic data is vital. Bioinformatics helps in understanding complex genetic traits and their relationships, forming the backbone of modern plant breeding strategies.
Adopting these technologies ensures that researchers can better tailor crops to specific environmental conditions. As these tools hone in on genetic nuances, the bar is raised in our pursuit of agricultural excellence.
Potential Impacts on Agriculture
The future of genotype research undeniably holds significant implications for agriculture.
- Increased Crop Yields: With a deeper understanding of genetic traits, researchers can select and breed plants that withstand stress, ultimately leading to higher yields.
- Nutritional Enhancement: Genetic insights facilitate the breeding of crops with improved nutritional profiles. For example, biofortified crops that address micronutrient deficiencies in certain populations can emerge confidently from rigorous research.
- Sustainability: Environmental pressures necessitate sustainable practices. By understanding genotypes, agriculture can shift towards crops that require fewer inputs, such as water and fertilizers, thus preserving natural resources.
- Pest and Disease Resistance: The ability to identify and enhance natural defenses within plants will reduce reliance on chemical pesticides. Improved resistance translates to healthier ecosystems and less harmful residue.
- Adaptation to Climate Change: Knowing how specific genotypes react to changing climates will guide the development of crops that flourish under new conditions, ensuring food security.
"The future of agriculture hinges on our ability to adapt and innovate through understanding the fundamental genetics of plants."
Case Studies in Plant Genotype
Exploring case studies in plant genotype provides a real-world lens through which we can examine the relevance and application of genotypic principles in plant biology. By analyzing specific instances where genotypes have been successfully utilized or have fallen short, we can glean lessons about the role of genetic make-up in shaping plant behavior, resilience, and adaptability in varying environments. It also highlights the complexities and nuances of working with plant genetics in agricultural contexts. These studies serve not only as tests of theory but also as blueprints for future endeavors in plant breeding and genetic research.
Successful Genotype Applications
One of the standout examples of successful genotype applications includes the efforts to enhance disease resistance in crops such as rice. In the 1970s, researchers identified a specific genotype in wild rice species that exhibited a robust resistance to a common fungal pathogen. Through selective breeding, they incorporated this genotype into cultivated varieties. This process didn't just improve yields; it saved countless farmers from total crop loss during outbreaks. The successful integration of these resistant traits into popular rice strains showcased the powerful potential of genotype in providing sustainable solutions to agricultural challenges.
Consider the case of genetically modified Bt cotton. Scientists inserted a gene from the bacterium Bacillus thuringiensis, which enabled the cotton plants to produce a toxin that affects specific insect pests. The result? A substantial decrease in the need for chemical insecticides. Farmers have reported increased crop yields, reduced environmental impact, and overall improved economic viability. Such applications demonstrate the nuances of genotype manipulation—wherein the understanding of genetic principles can alleviate pressing agricultural issues.
Key aspects contributing to these successes include:
- Thorough research: Understanding how specific genotypes interact with environmental factors.
- Stakeholder collaboration: Engaging with farmers and local communities to tailor solutions to their unique challenges.
- Ongoing assessment: Monitoring the impacts of these applications over time to ensure they continue to meet agricultural needs.
"Through case studies, we find the pulse of genotype's real-world applications, turning theory into tangible results for farmers."
Lessons Learned from Failures
While the narrative of success is inspiring, it is equally pivotal to consider lessons learned from failures in the realm of plant genotype research and application. One notorious example is the drastic failure of genetically modified crops in certain environmental conditions. In the mid-2000s, the introduction of drought-tolerant corn hybrids in regions with unpredictable water availability spiraled into disarray as the crops did not perform as expected under true drought stress. This highlighted the over-reliance on genotypic traits without a comprehensive understanding of environmental dynamics.
Another case involved the initial deployment of proprietary seeds which promised high yields but resulted in significant crop loss due to unforeseen pest attacks. The genotype wasn’t resilient enough against local insect populations, showing that predicting pest behavior is as crucial as selecting genotypes. This teaches us that understanding micro-environmental factors and local ecosystem interactions is key in selecting and applying genetic traits.
Some important takeaways from these experiences are:
- Comprehensive assessments: Before any genotypic application, thorough testing in varying conditions is essential.
- Adaptability: Breeding programs should incorporate flexibility to adjust to new environmental challenges as they arise.
- Community involvement: Engaging local farmers and researchers fosters an exchange of knowledge that can lead to more sustainable practices.
In summary, case studies in plant genotype unveil a significant tapestry of successes and failures that inform our understanding of how genetics impacts agriculture. By scrutinizing successful genotype applications alongside lessons learned from failures, we sharpen our insights into optimizing genetic strategies for sustainable farming.
Comparative Genomics in Agriculture
Comparative genomics has emerged as a vital component in the realm of agriculture, fostering a deeper understanding of the genetic blueprint across different plant species. This discipline examines the similarities and differences in genetic material, offering insights that can lead to significant advancements in crop science and genetic engineering. By comparing genomes, researchers can identify valuable traits such as disease resistance, drought tolerance, and nutritional content that may be present in one species but absent in another.
The importance of comparative genomics lies in its ability to streamline breeding efforts. Through detailed genetic analysis, scientists can pinpoint specific genes associated with desirable traits. This targeted approach not only saves time but also enhances the precision of breeding programs. By utilizing genomic information, agronomists can develop new varieties that are better suited to specific environmental conditions, thus optimizing yield and sustainability.
Additionally, the insights gained from comparative genomics can inform conservation strategies. By understanding the genetic diversity within and between species, it's possible to conserve endangered plants more effectively. Preserving genetic diversity ensures resilience against environmental changes and plant diseases, providing a buffer for future agricultural challenges.
Analyzing Different Plant Species
When analyzing different plant species, comparative genomics provides a treasure trove of data that can illuminate both evolutionary pathways and functional traits. For instance, studies comparing the genomes of domesticated crops like maize and its wild relatives reveal how selection pressure influenced genetic changes over time. Such analyses not only uncover which genetic variations were favored through domestication but also guide breeders in selecting traits that bolster agricultural productivity.
"Comparative genomics is like holding a genetic roadmap of the plant kingdom; it helps us navigate through both known and unknown territories of plant traits."
In practical terms, analyzing different species can lead to enhanced cross-breeding strategies where genes known to confer specific advantages in one plant are introduced into another. This cross-species examination fosters innovations such as hybrid crops, which combine the best traits from multiple sources, creating plants that are both resilient and productive.
Cross-Species Genotype Insights
Cross-species genotype insights allow for a revolutionary perspective on plant genomes, drawing connections between diverse agricultural species. By studying the genetic sequences across various plants, researchers can gain an understanding of how certain traits have evolved in response to similar environmental pressures. For example, insights from the genomes of leguminous plants have revealed mechanisms for nitrogen fixation that could potentially be harnessed in other crop systems, improving soil fertility and reducing fertilizer dependence.
Moreover, these insights can unveil integrative strategies for pest and disease resistance. For instance, if a genome study identifies a resistance gene in a wild species of tomato, breeders may utilize that information to enhance the resilience of cultivated varieties. This enables a proactive approach to tackling agricultural threats, paving the way for more sustainable practices.
Closure: The Role of Genotype in Future Agriculture
Understanding the role of genotype in plants holds key significance for the future of agriculture. This knowledge not only enhances the approach to crop improvement, but it also fortifies the entire agricultural framework against environmental challenges and market demands. Genotype serves as the underlying foundation that determines how plants interact with their surroundings, emerge under selective breeding, and adapt to various stressors such as drought, soil salinity, and pests.
As agricultural practices evolve, understanding genotype is critical for creating resilient crop varieties that withstand the rigors of climate change. The integration of advanced biotechnological tools has provided researchers and farmers alike with an arsenal of methods to enhance traditional breeding approaches. This understanding can lead to improved yield, better quality products, and even a more sustainable agricultural system.
Summary of Key Insights
- Genotype and Phenotype: The genotype offers insights into potential traits that can be expressed under specific environmental conditions, explaining the divergence in crop performance.
- Technological Advancements: The rise of technologies such as whole genome sequencing and SNP analysis are revolutionizing how agronomists and farmers examine and apply genotype information.
- Environmental Interactions: Understanding how different genotypes perform in diverse environmental contexts enables targeted strategies in breeding and selection, enhancing adaptability.
- Sustainability Focus: Adopting genotyping technologies supports sustainability goals by optimizing resource use and developing crops that require fewer inputs.
Through these insights, it's clear that a thorough grasp on genotype allows for informed decisions in agricultural practices.
Final Thoughts and Recommendations
It’s essential for agricultural stakeholders – from farmers to researchers – to harness the insights provided by genotype research. Here are a few recommendations:
- Invest in Research: More funding should be allocated to genotype research to refine crop resistance and yield traits.
- Adopt New Technologies: Farmers should consider incorporating modern genotyping technologies into their practices. The initial costs might be high, but the long-term benefits can outweigh these upfront expenses.
- Collaboration: Partnerships between academic institutions, agricultural organizations, and farmers are vital for disseminating the knowledge gained from genetic studies effectively.
- Focus on Education: Continuous training programs should be established to educate stakeholders on the latest advancements and applications of genotype knowledge.
In uncharted waters of agricultural innovation and sustainability, genotype is not just an aspect to observe; it’s a necessary tool for guiding us toward a more efficient and resilient food production system. By approaching the future of agriculture with a clear understanding of genotype, we can build a more robust framework that benefits everyone involved.